How to put life on hold… or die temporarily
- Death is a process with a complex definition, characterised by a number of different elements.
- The living world is full of examples that overturn our binary representation.
- Seeds, for example, can remain in a state of inactivity, known as dormancy, until the right external conditions trigger their germination.
- Dormancy or cryptobiosis, a form of temporary death, can become veritable time capsules, giving “organisms remarkable longevity.
- These different forms of slowed life open up a debate on our definition of death and the world around us.
The heart stops, there is no brain activity, the body cools down and, finally, the molecular activity within each cell disappears. Although not simultaneous, human death is marked by several characteristic elements. However, determining whether an organism is dead or alive is not always straightforward. There are complex clinical situations: animals, for example, practice thanatosis, or death simulation, to deter predators. Many organisms can go through states that challenge our binary perception of life and death.
In your kitchen cupboards you may find rice, lentils, nuts, potatoes, onions, apples… All these structures are of plant origin. In other words, they were once alive. But which ones are still alive? In some cases, the answer is obvious: a stalk protruding from a potato fillet or a sprout piercing the skin of an onion are not so subtle clues. There is life in your cupboards. But it’s not always so clear-cut: how can you tell the difference between a dead and a living lentil?
Seeds are reproductive structures, containing an embryo and nutrient reserves sheltered by a protective integument. They are capable of remaining in a state of apparent inactivity until external conditions (temperature, light, humidity, etc.) trigger germination. In the meantime, they show no signs of life, but this does not mean they are dead. In fact, they are in an extremely slowed-down state of life known as dormancy. And this state is reversible: if you place lentils on wet cotton wool, they will probably end up germinating. But there’s no point in trying the same thing with white rice. Those seeds have been hulled and only the nutrient tissue they contained has reached your kitchen.
Slowing life down to a standstill
Dormancy is a widespread phenomenon in the natural world. In some organisms, it is systematic and genetically programmed, while in others it is triggered only when living conditions become too unfavourable. The term diapause or quiescence is also used to describe certain forms of life slowing down. Like seed plants, various mammals can, for example, put their reproduction on hold, with females saving embryos without immediately implanting them in their uterus. This process, known as embryonic diapause1, makes it possible to adapt life cycles to the resources available – which vary according to the season – and to ensure the best possible conditions for the offspring.
In some organisms, the metabolism doesn’t just slow down: it stops. These organisms are said to be in cryptobiosis, literally “hidden life.” They are not dead, since this state is reversible, but they are no longer obviously alive. Cryptobiosis can therefore be considered as life in a latent state, a form of temporary death, or as a third state, different from both life and death2. In fact, the physiology of organisms in cryptobiosis is profoundly altered.
There are several forms of cryptobiosis, linked to different extreme conditions. The most extensively studied is anhydrobiosis. Anhydrobiosis is characterised by the loss of almost all the water in an organism, which is essential for maintaining its integrity at cellular and bodily level3. Local replacement of water, transition to a vitrified state or specific protection of certain compounds, various molecular adaptations make it possible to tolerate this drastic change4. As a result, when they are rehydrated, anhydrobiotic organisms can come back to life, or revivify. Understanding the mechanisms involved in this phenomenon could be a source of innovation for all processes for preserving biological structures by drying or freezing, both in medicine and the food industry.
The inventiveness of micro-organisms
Cryptobiosis exists on every branch of the living tree. Animals are capable of it, notably rotifers, nematodes and the famous tardigrades5. But plants are also affected, such as mosses and certain ferns. The list extends to lichens, fungi and many unicellular, eukaryotic and prokaryotic organisms. Many micro-organisms can also form resistance structures, more or less dehydrated, whose metabolic activity is slowed or even stopped.
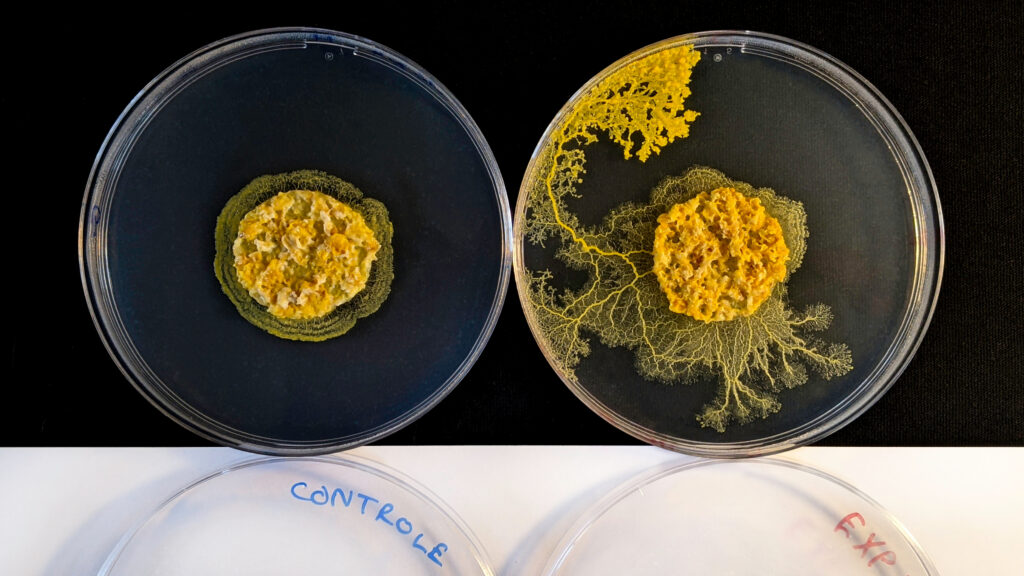
Some fungi and myxomycetes, such as the blob Physarum polycephalum, survive difficult periods in the form of desiccated sclerotia. Bacteria can divide asymmetrically to produce endospores that are extremely resistant, including to heat and antibiotics. Many protists, unclassifiable unicellular eukaryotes that are neither animals, plants nor fungi, form cysts. Resistant to cold and desiccation, these structures enable many parasitic species to spread. Similarly, viral particles are inert in the external environment until they encounter a cell to be infected.
Whether this is dormancy or true cryptobiosis with cessation of metabolism (which is not easily determined in practice6), these astonishing states can become veritable time capsules, particularly when placed in favourable conservation conditions. Cysts have been brought back to life after spending a hundred years in the sediments of a Swedish fjord7 or at the bottom of the Baltic Sea8. Mosses have been revived after a thousand years in the Antarctic permafrost9. In the Arctic, nematodes that emerged from 30,000 to 40,000-year-old permafrost have been revived in the laboratory10, as have parthenogenetic rotifers that have been buried for around 24,000 years11. The oldest viruses that are still infectious, taken from frozen Siberian soil, are giant viruses that infect amoebas and are close to 50,000 years old…
Questioning our definitions
The existence of different forms of slowed or arrested life has given rise to debate among specialists: where does dormancy end and cryptobiosis begin? Is the latter just an extreme form of the former? Which structures fall into which categories? The world around us is in fact a continuum, in which it may seem futile to try to distinguish clear-cut categories. And this also applies to the notions of life and death. Whether we favour a definition based on functions, structures, physical chemistry or philosophy, extreme cases are valuable food for thought.
Can we say that microscopic animals that have survived for tens of thousands of years in frozen ground have “lived” there? Did they live for an extremely long time, were they temporarily dead, or did they experience a state that is neither life nor death? These questions seem to be taken from works of science fiction, involving long interstellar journeys, but they are being asked by organisms that live on our planet today. And for the moment, there is no consensus on how to answer them.